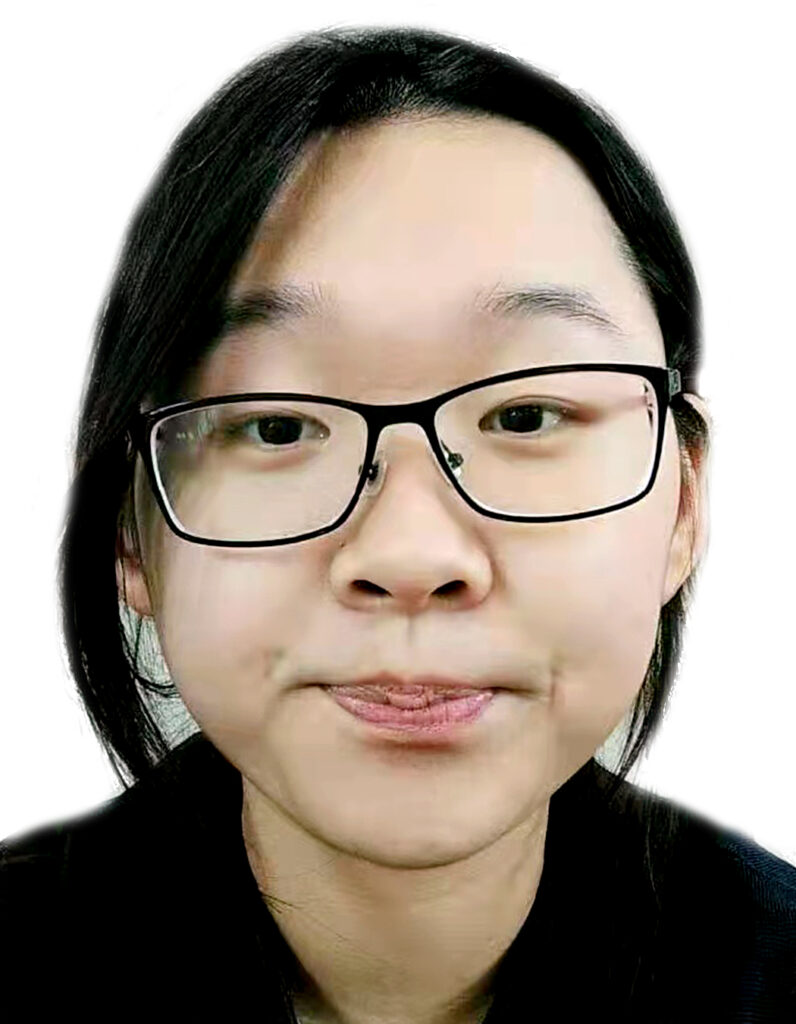
ESR: Bohan Zhang
PI: Gerhard Gompper, Dmitry Fedosov
Forschungszentrum Juelich
Research topics:
Bacteria Aggregates, Swarming, Collective Behaviour, Squirmer, Numerical Simulation.
Biofilm is a complicated structure where bacteria move collectively and form highly stable and motile aggregates; we call the collective locomotion of bacteria inside aggregates swarming. We will run a large number of numerical simulations of swimmers and we represent the swimmers with a
well-developed model ‘squirmers’. By examining different parameters controlling the behaviour of a single squirmer, such as the strength of inter-bacteria propulsion, the shape and the density of bacteria, etc., we expect to understand the formation and motility of bacteria swarming aggregates.
Goals:
- To understand the collective behaviour of bacteria swarming in a detailed level.
- To illustrate the different aspects contributing the formation of motility of swarming aggregates.
- To format the connection between the motility of swarming aggregates and the internal properties of individual swimmers.
Challenges: The theoretical model has only been developed for single-layer squirmers so far. However, to achieve the goal of the project, we need to include the formation of multiple squirmer layers and different squirmer
swimming directions in our new model.
Bohan Zhang is working toward the direction of hydrodynamic simulations of multiple microswimmers, which mimic collective behaviour of bacteria within a biofilm. She has started with a literature review on motility-induced phase separation in active suspensions and mesoscopic hydrodynamic methods, such as dissipative particle dynamics. Then, she has familiarised herself with our parallel simulation code, which enables simulations of multiple swimmers represented by a squirmer model. Bohan has performed a number of simulations with a single squirmer, in order verify its swimming speed and generated flow field against available theoretical predictions. Soon, she is going to perform simulations with multiple squirmers, in order to study their collective behaviour.
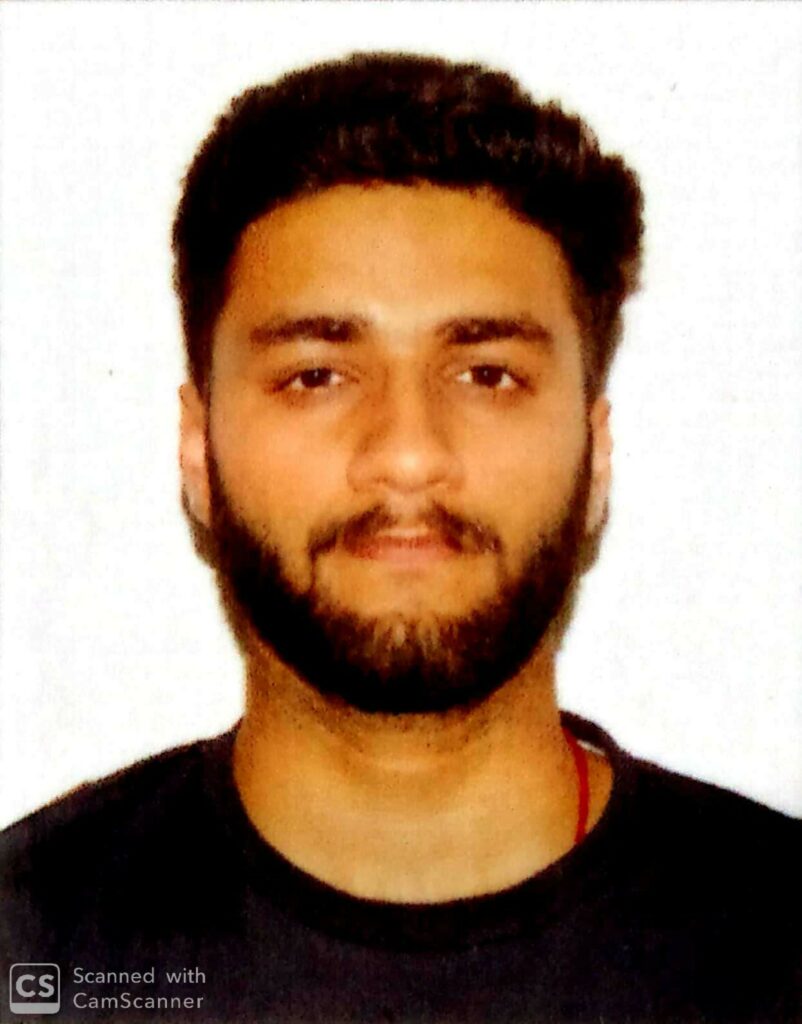
ESR: Shubham Anand
PI: Gerhard Gompper, Jens Elgeti
Forschungszentrum Juelich
Research topics: Multi Particle Collision Dynamics, Bacteria, Cilia, Flagellar Mechanics, E. coli, Beat pattern, Cilia Synchronisation, Brownian Dynamics, Numerical simulations.
Cilia and (eukaryotic) flagella are ubiquitous and they also preserve the well-conserved structures. They consist of a filamentous structure composed of microtubules in a ring-like arrangement, and dynein molecular motors which slide these microtubules, allowing flagella motion. Due to the sliding behaviour, there is bending, beating, and propulsion in viscous hydrodynamic medium. Our aim is to advance our understanding of the origin of how motors coordinate. Based on the previously developed single-cilium or single-flagellum model, we will look into the swimming behaviour of multi-flagellated microswimmers (e.g. algae, Volvocales) as well as their cilia synchronisation in response to external perturbation-like imposed flow, neighbouring microswimmers, or confinement.
Goals:
- To establish a theoretical model for the autonomous beating of cilia and eukaryotic flagella.
- To compare the simulation results of the beating pattern of a single-cilium with experimental observation, therefore, to determine the parameters required for a realistic beating.
- To study the pheonomona of Emergent Metachronal Waves and collective swimming of multi-ciliated microswimmer and thus compare with experimental results.
I am currently learning and simulating some active systems with already studied methods in Molecular Dynamics. Also, I will be a mentor in a biophysics workshop in Paris, where I will teach students about the physics of microswimmers by modeling Active Brownian Particle Dynamics with various systems.
Challenges: Because I have just begun my Ph.D., I am still on my way to getting familiar with different methods for numerical simulations, parallel programming, etc. Also, the optimisation of code in python and CPP is also another obstacle for me.
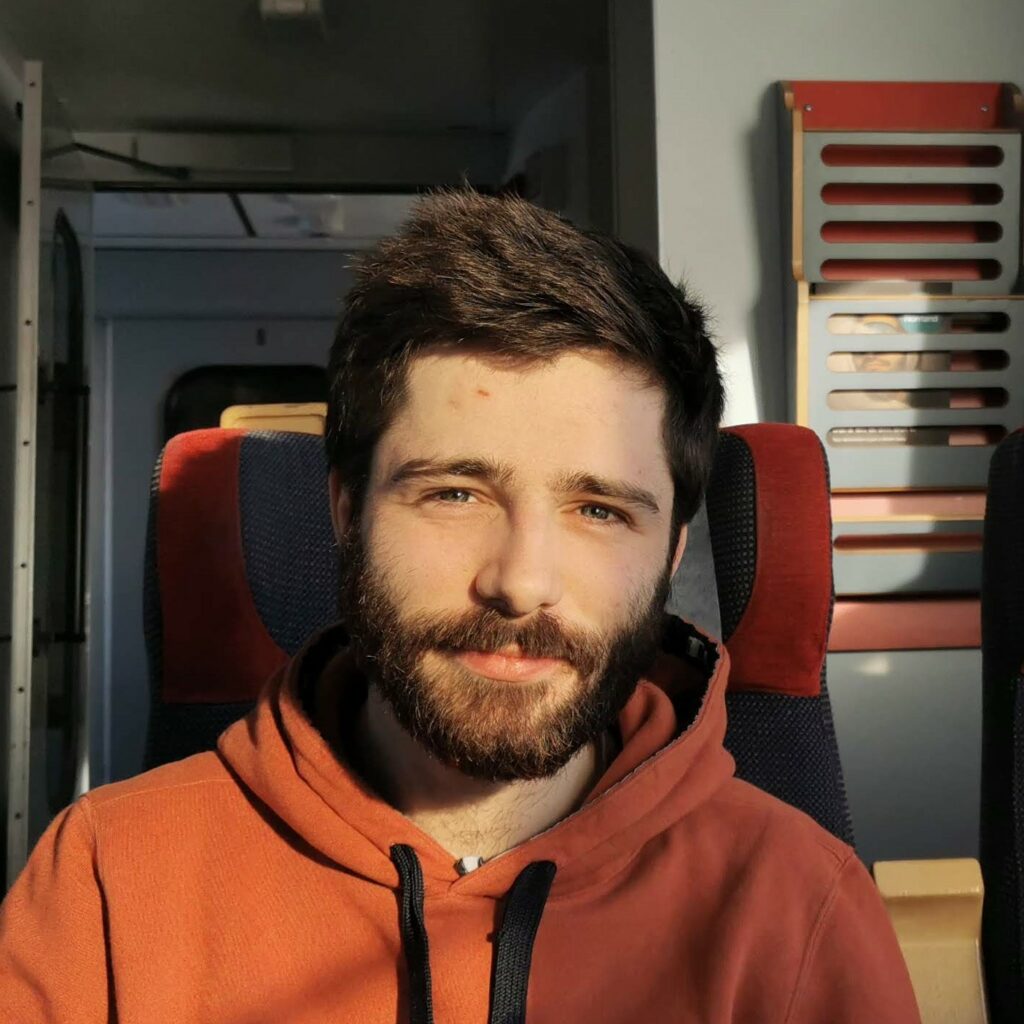
ESR: Luc Zorrilla
PI: Marco Polin
IMEDEA, Mallorca, Spain
Research topics: Motile flagella, Chlamydomonas,
Axoneme, Viscoelasticity, Active Rheology.
Cilia and flagella are slender organelles common to a wide spectrum of eukaryotes, both marine and terrestrial. Their deformations either passive or active underpin essential processes, from mechanical sensing and locomotion of aquatic microorganisms to the generation of feeding currents in corals. Autonomous ciliary beating is particularly important. It stems from the coupling between the passive properties of the internal structure of the flagellum and the activity of hundreds of molecular motors within it. The detailed mechanism leading to beating, however, is currently not well understood. To progress, it is critical to have a precise grasp of the visco-elastic properties of the axoneme. So far, experiments have only probed static elasticity. This project aims to use an active-rheology approach to measure directly both viscous and elastic components of flagellar mechanics, to develop the required experimental and data analysis techniques, this could lead to an improved, experimentally-tested model of the axoneme.
Our goal is to address the following questions:
- How do the elastic properties of the axoneme change under shear? (e.g. shear-thinning or shear-thickening)
- What is the magnitude of the internal viscosity in the axoneme?
- How do the mechanical properties of basal bodies and associated fibres impact the biomechanic response of the flagellum?
- What is the role played by constitutive axonemal structures (e.g. nexin links, radial spokes) and dynein cross-bridges in the biomechanics of the axoneme?
- How do we model the axoneme in a way consistent with the dynamic properties characterised experimentally?
I am currently working on three things:
- Making a pipeline for image and data analysis from experiments. I am trying to isolate the flagella in videos of fixed cells that have beating flagella.
Challenges: there are many possible options to analyse the image. The decision to making the right choice of analysation method compared to up-coming experimental data is a challenging. - Making a review of the viscoelastic properties of the axoneme in motile and primary cilia.
Challenges: I am still on my way to getting familar with the field of research. - Understanding recent models of the axoneme and eventually running simulations of these models.
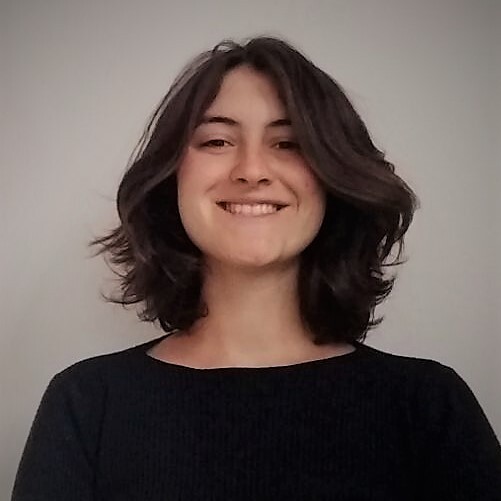
ESR: Medea Zanoli
PI: Idan Tuval Gefen
IMEDEA Palma de Mallorca
Research topics:
Parasitism, Population-scale Dynamics, Predator-prey Interaction, Mutual Sensing Mechanism, Collective Behaviours.
The equilibrium of the marine ecosystem relies on a complex network of interactions between different species, which regulates the proliferation
or decline of populations. Parasitism might represent a key top-down control for the dinoflagellate populations of the Mediterranean Sea, affecting its temporal dynamic just as much as the chemical and physical factors typically considered. In this research project, we focus our interest on the interaction between the widespread dinoflagellate species Alexandrium minutum and its motile flagellated parasite Parvilucifera sinerae whose life cycle seems to have well adapted to that of its seasonally blooming host, bound together by a predator-prey temporal dynamics.
Our objective is to unravel the rules of the interaction between the parasite and its host, and the surviving strategies deployed by the parasite to
achieve a successful infection cycle. We will tackle open questions such as:
- How does the replicating and densely packed parasite population manage to find an escape route from the confined environment of the sporangium (the empty shield of the infected host)?
- Do collective behaviours play a role in the survival of parasite populations, both inside the sporangia and outside in an open water?
- Which mutual sensing mechanisms are deployed by the parasite to detect a swimming-by host? Can the host try to actively avoid its predator?
We will tackle these issues from both an experimental and theoretical perspective, borrowing techniques from micro-fluids mechanics and microscopy to set up controlled laboratory conditions to reproduce the natural ones.
I am currently working on three side projects:
- The role of Dissolved Organic Carbon (DOC) as grazing infochemicals for microzooplankton [collaboration with Q. Guell, ICM Barcelona]
We are collaborating with a team of biologists and chemists from ICM in Barcelona to develop an automatized method for doing video tracking of a large set of chemotaxis experiments. From these videos we intend to answer the questions such as does the chemotactic response of the organism depends on the concentration of chemical substance and in which extent; does the presence of the chemical subtance change with the swimming behaviour of the organisms (in terms of velocity distribution, angle distribution and shape of trajectory, etc.) ?
Challenges:
Setting the right parameters to automatically detect only the cells on the microscope focal plane. - The role of physical forcings in the temporal dynamics of near-shore algal blooms. [collaboration with Gotzon Basterrechea Oyarzabal,
IMEDEA, Palma de Mallorca]
We are collaborating in the SifoMED project by developing a simple theoretical model to describe the accumulation-relaxation pattern of near-shore biomass during algal blooms, taking into account very simple physical forcing (wind advection and freshwaters pulses). - Getting Alexandrium minutum to stay alive in laboratory conditions:
I am getting familiar with culturing the microorganisms that we will need to run the experiments.
Challenges: They keep dying.
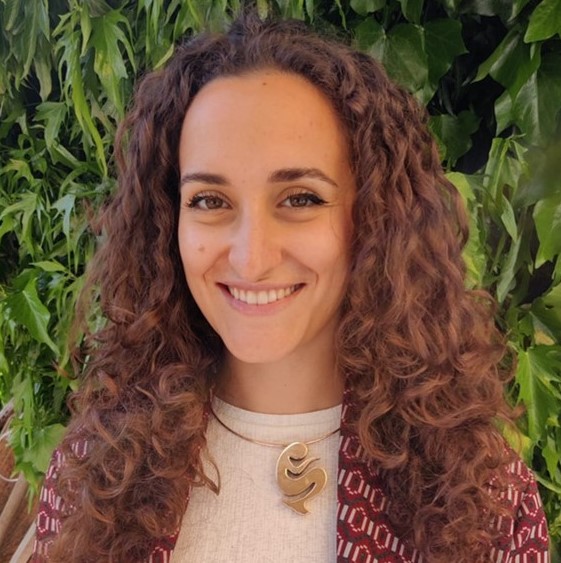
ESR: Federica Miano
PI: Thomas Kiørboe
Centre for Ocean Life, DTU Aqua, Technical University of Denmark
Research topics:
Experimental Plankton Ecology, Fluid Dynamics of Flagellate Motility, Ecology and Evolution of Flagellate Foraging.
Flagellates represent highly relevant eukaryotic species as they are found among all the branches of the tree of life with a huge morphological and behavioral diversity. Also, they play a crucial role in the marine microbial food web by feeding bacteria and other picoplankton, by their photosynthetic activity, and by themselves being grazed by rheotactic predators. Therefore, they have evolutionarily developed different feeding and predator avoidance strategies to find an equilibrium between resource acquisition and predation risk.
Our goal is to describe these trade-offs quantitatively. Because the degree of success in eating without being eaten is the key to understanding the functioning of predatory flagellates. We will select representative species from different branches of the tree to look at their behaviours as both prey and predators and address the following questions. We aim to answer these questions by combining experimental observations with theoretical approaches and Computational Fluid Dynamics (CFD) modeling:
- How can flagellates sense predators and perform rapid escape jumps to avoid them?
- How do diverse flagellar arrangements, kinematics and beat patterns result in different resource acquisition modes?
- How are foraging-predation risk trade-offs constrained by species diversity?
We are currently looking at the behaviours called escape jumps that species with diverse flagellar arrangements perform. We are using high-speed video microscopy and microfluidics to examine different perspectives, such as these species’ ability to perceive predators, the characteristics and threshold magnitude of fluid and mechanical signals, and the mechanism allowing these species to increase their escape speed by more than one order of magnitude.
Challenges: when analyzing escape events, we have to take into account that the organisms go out of focus during the jump to have unbiased measurements. A future goal is to develop a 3D tracking technique.
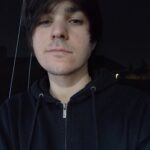
ESR: Riccardo Foffi
PI: Roman Stocker
ETH Zurich
Research topics:
Bacteria-phytoplankton Interactions, Chemotaxis, Cell Tracking, Bacterial Behaviour in Oligotrophic Environments.
Marine bacteria mediate the major biogeochemical cycles in the ocean and take part in important symbiotic interactions. From their point of view, the environment is a heterogeneous landscape of chemical hotspots, which they can locate and exploit using chemotaxis.
Our goal is to advance the understanding of chemotaxis on different levels and answer the following questions, using a combination of theoretical modeling, simulations, and experiments:
- How do environmental conditions like turbulence, noise and nutrient availability affect foraging strategies and decision-making of marine bacteria?
- What are the limits of sensing?
- How do interactions between individual organisms, mediated by chemotaxis, shape the ecological landscape of the ocean?
I am currently working on two projects:
- microArgos [with François Peaudecerf]
A low-resolution fluorescence-based tracking system designed to follow individual bacteria over long timescales (~15 hours) and distances (~1cm). Inspired from the Argos satellite system used to track movements of animals over the whole globe (e.g. migratory routes).
Challenges:- Optimization of lighting/camera settings, data acquisition setup
- Tuning of tracking algorithm to deal with the particular data acquisition format (non-homogeneous spacing in time and spatial overlaps)
- Effect of chemotaxis on encounter rates [with Jonasz Slomka, François Peaudecerf]
We developed a simple theoretical model to estimate the chemotactic contribution to encounter rates between bacteria and nutrient-leaking sources (e.g phytoplankton cells). We are now running numerical simulations of chemotaxis in the presence of sensing noise to make predictions about ecologically-relevant encounters in marine environments.
Challenges:- Understand and model the effect of noise on chemotactic behavior
- Understand ecological implications
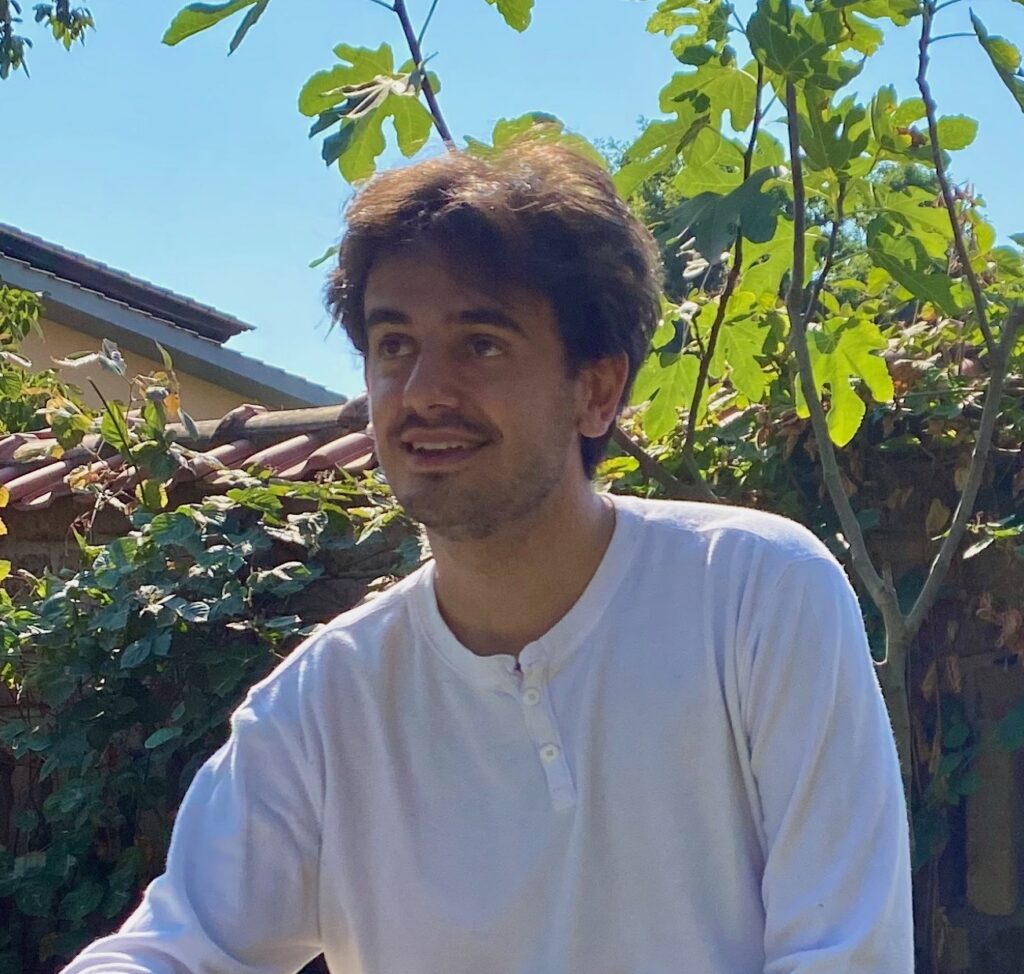
ESR: Tommaso Pietrangeli
PI: Cécile Cottin-Bizonne, Christophe Ybert, François Detcheverry
Université Claude Bernard Lyon 1
Research topics:
Magnetotactic Bacteria, Active Transport in Complex Environments, Bacterial Motility.
Many bacteria have the ability to move through their environment to meet their needs whether for nutrients or oxygen. It is striking that bacteria have evolved a rich repertoire of swimming modes, but the question of what are the benefits of a particular swimming pattern is still open. In addition, bacteria often have to move under mechanical stresses, such as hydrodynamical or geometric constraints. Our understanding of their movement under such constraints is currently limited. We plan to explore these issues from a physical point of view, using both a theoretical approach and an experimental study of magnetotactic bacteria; they are micro-swimmers that naturally possess a magnet within their body, and can thus be steered by a magnetic field.
Goals:
- To characterise experimentally the movement of a novel strain of magnetotactic bacteria (MTB-SS5) with respect to different mechanical constraints.
- To investigate numerically which are the optimal swimming strategies for a driven system in a complex environment.
I am currently working in parallel on two projects:
- On the numerical side, I have developed a code that simulates a broad range of bacterial swimming strategies. I am currently implementing the different motilities in complex environments, such as porous media.
- (In collaboration with CEA Cadarache) On the experimental side, I am learning the protocol for the growth of the SS5 novel strain of magnetotactic bacteria and I am working on suitable micro-fluidic devices to study future systems.
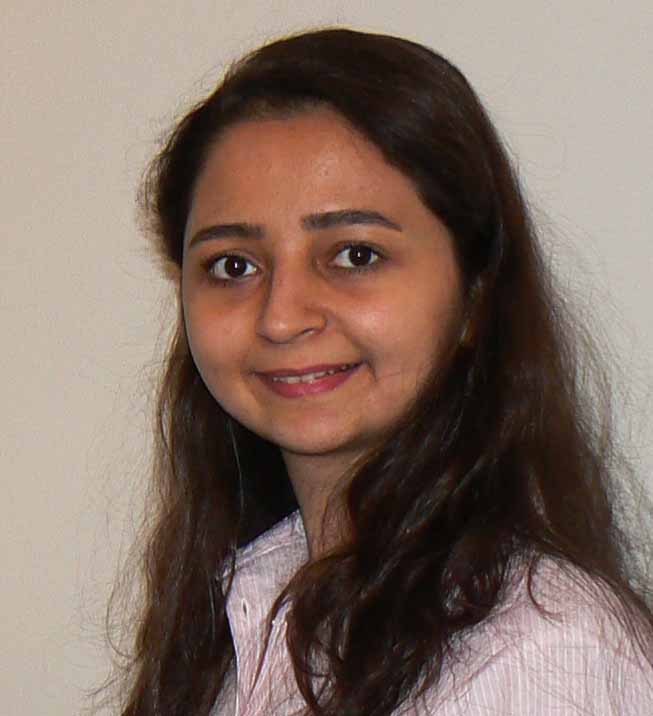
ESR: Narges Jamshidi Khameneh
PI: Markus Engstler
University of Wuerzburg
Research topics: Trypanosome, Microfluidic, 3D Microscopy
African trypanosomes are unicellular parasites that infect animals and human hosts and cause deadly diseases. They require a set of particular capabilities to adapt to their environments in the tsetse fly and their mammalian hosts during their life cycle. Each stage of the parasites’ developmental cycle performs individual tasks. In each stage, trypanosomes adjust with noticeable changes in morphology and motile behaviour. Further, geometric parameters and flow properties of their living environment influence their motion patterns.
Our goal is to describe the motile behaviour of various trypanosome stages under the influence of environments acting as confining tissues, i.e. cavities and channels that influence the motility of the flagellate swimmers directly or by defining hydrodynamic properties of their proximity. We aim to analyse this influence by mimicking natural environments, as characterised by microscopic 3D maps. We plan to establish surroundings in vitro by using various materials and microfluidic channels which allow us to quantitatively perform 4D analysis of flagellar dynamics to explain single cell and collective motion patterns.
Currently working on:
As a physicist, I am currently learning cell biology and cell culture techniques. I am performing preliminary microscopy with different cell lines, in order to establish standardised data sets on the parasites’ motion behaviour, at specific resolution and with different physicochemical properties of the surrounding environment.
Challenges:
It is quite hard to maintain well-defined conditions for living cells and their environments in culture, especially during microscopic analysis, while allowing analysis of flagellar dynamics under high resolution.
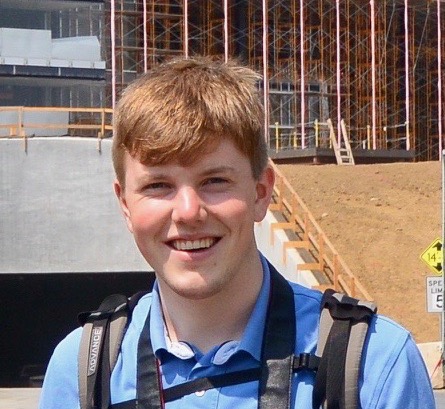
ESR: Morten Kals
PI: Pietro Cicuta, Allen Donald
University of Cambridge
Research topics: Single-cell Time-lapse Microscopy,
Bacteria, Antibiotic Susceptibility Testing, Automation, Cell Segmentation
Antibiotic resistance is a growing issue in healthcare and will be one of our most challenging healthcare problems. Many solutions are needed, one of which is to develop tools for more effectively targeting bacterial infections using the existing antibiotics.
Goals:
Our goal is to develop a platform for higher throughput antibiotic susceptibility testing. The bacterial sample will be placed on a series of 96 agarose pads with different antibiotics presenting at varied concentrations. They will be confined to a single imaging plane using a glass coverslip and imaged for four hours under a microscope. An image processing toolchain will be employed to analyse the resulting images and determine what conditions inhibit the growth of bacteria.
I am currently working on developing the tooling for capturing and analysing images. This includes:
- Sample platform development and testing.
- Writing Scripts to get the microscope to image appropriately with sufficient time-resolution.
- Image analysis pipeline to robustly output statistics from imaging positions.
Challenges: In the step of writing scripts, due to the large size of the sample slide, it is especially difficult to ensure the sample remains in focus for all imaging locations.
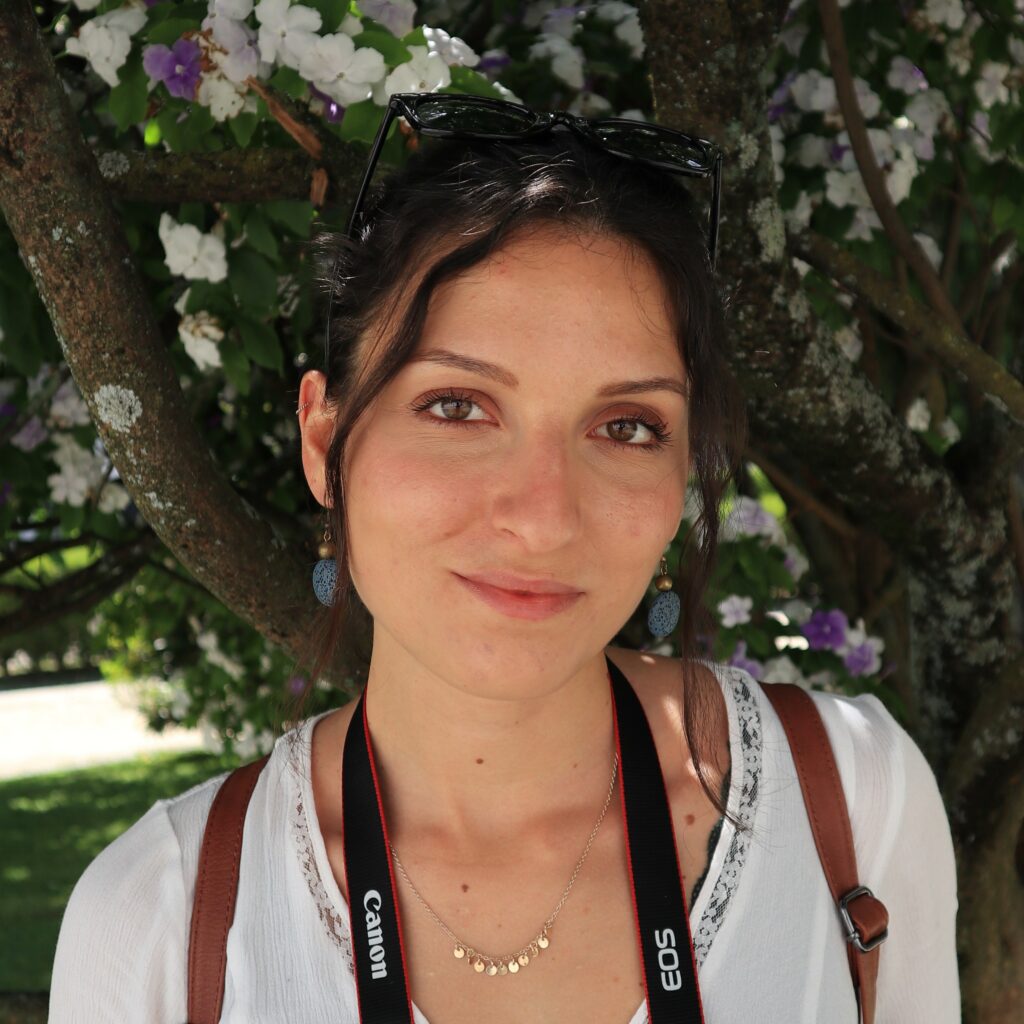
ESR: Erika Causa
PI: Pietro Cicuta
University of Cambridge
Research topics: Airway Motile Cilia,
Cilia Collective Behavior and Synchronisation,
Fluid Transport in the Airway Surface Liquid,
Bacterial Infection.
In the human respiratory tract, the coordinated beating motion of cilia underpins an essential defense mechanism: mucociliary clearance. Here, the multi-ciliated epithelium is covered by a layer of mucus that traps inhaled pathogens and environmental debris. The mucus is then unidirectionally transported out of the airways due to a fluid flow generated by the synchronised beating of cilia. Our aim is to answer open questions concerning the cilia-driven fluid flow and cilia alignment in ciliated epithelia.
Goals:
- To characterise the fluid dynamics in the airway surface liquid (ASL) with particular focus on the periciliary layer (liquid layer in which cilia are immersed).
- To investigate how the motility of pathogenic bacteria is affected by the presence of cilia-driven flow.
- Using induced pluripotent stem cells (iSPCs) – derived airways epithelia as a model system for investigating how cilia alignment and orientational order develops.
I am currently working on two sub-projects:
- Determine the velocity profile of fluid in the periciliary layer (PCL) with a new technique that allows us to track the fluid in a highly packed area without the use of colloids. Then Correlate the transport in the PCL with that in the mucus layer.
Challenges: Improving the imaging technique for longer experiments; finding good clear areas where we could perform analysis; fitting the data with existing models.
- (In collaboration with Cambridge Stem Cells) Assess synchronisation, coverage and ciliary beating frequency in iSPCs-derived epithelia and compare that information to the one collected with primary cells.
Challenges: To have a high coverage (not only cilia patches) and coordinated cilia movement.
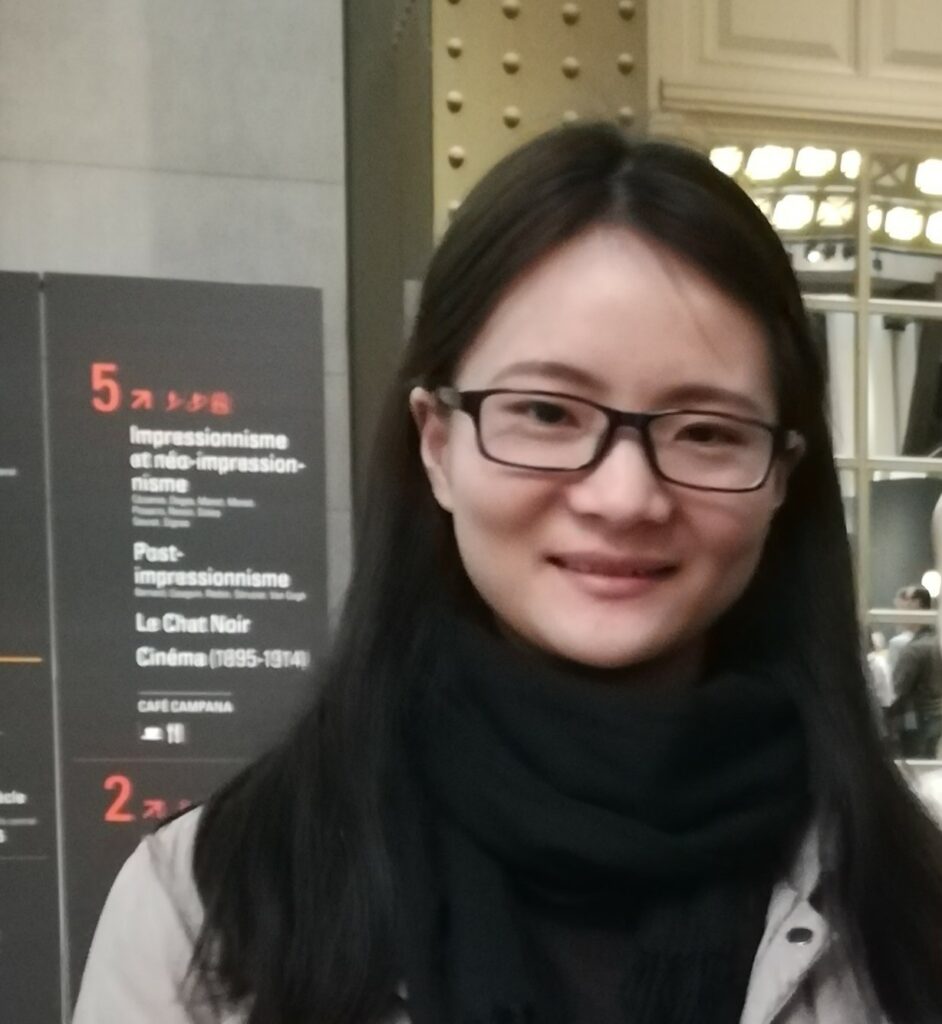
ESR: Peixin Zhang
PI: Anke Lindner, Eric Clement
ESPCI-Paris
Research topics: Bacteria, Active Transport, Hydrodynamic Dispersion, Tracking, Confined and Complex Environments
Bacteria are known to interact with flow and bounding surfaces leading to phenomena called bacterial rheotaxis or persistent upstream swimming. In disordered and complex environments, the transport of bacteria is also the key to many processes such as bioremediation or bacteria assisted drug delivery. To elucidate the combined role of flow and the presence of surfaces on bacterial transport, we will observe individual trajectories and bacteria dispersion using E coli bacteria in confined microfluidic channels with different complex channel geometries and complex carrier fluids.
Goal:
- To observe and characterise individual bacteria trajectories and global transport properties in complex confined environments.
- To Visualise the 3D trajectory and the flagella bundling and unbundling dynamics in parallel to understand the link between bacteria transport and run and tumble statistics
- To explore the transport of bacteria in 3D light transparent porous media with tunable properties.
I am currently working on:
- Characterise the transport and anomalous distribution of E.coli (RTPs) in geometrically symmetric microfluidic channels such as funnel geometry. The bacterial concentration and concentration difference as a function of flow rate are characterised up and downstream of the funnel for different channel confinements. Individual bacteria trajectories are analysed. Then the transport and dispersion in rectangular cells at different confinement heights will be considered using the Lagrangian tracking technique.
- Porous media fabrication and parameter optimisation: Firstly test has been performed to fabricate pillar arrays inside microfluidic channels using a microscope-based projection lithography technique and I am currently designing optimal array sizes for the experiment.